Planetesimal rings as the cause of the Solar System’s planetary architecture
DeMeo, F. E. & Carry, B. Solar System evolution from compositional mapping of the asteroid belt. Nature 505, 629–634 (2014).
Kruijer, T. S., Kleine, T. & Borg, L. E. The great isotopic dichotomy of the early Solar System. Nat. Astron. 4, 32–40 (2020).
Grewal, D. S., Dasgupta, R. & Marty, B. A very early origin of isotopically distinct nitrogen in inner Solar System protoplanets. Nat. Astron. 5, 356–364 (2021).
Brasser, R. & Mojzsis, S. J. The partitioning of the inner and outer Solar System by a structured protoplanetary disk. Nat. Astron. 4, 492–499 (2020).
Birnstiel, T., Klahr, H. & Ercolano, B. A simple model for the evolution of the dust population in protoplanetary disks. Astron. Astrophys. 539, A148 (2012).
Walsh, K. J., Morbidelli, A., Raymond, S. N., O’Brien, D. P. & Mandell, A. M. A low mass for Mars from Jupiter’s early gas-driven migration. Nature 475, 206–209 (2011).
Raymond, S. N. & Izidoro, A. Origin of water in the inner Solar System: planetesimals scattered inward during Jupiter and Saturn’s rapid gas accretion. Icarus 297, 134–148 (2017).
Huang, J. et al. The Disk Substructures at High Angular Resolution Project (DSHARP). II. Characteristics of annular substructures. Astrophys. J. Lett. 869, L42 (2018).
Dullemond, C. P. et al. The Disk Substructures at High Angular Resolution Project (DSHARP). VI. Dust trapping in thin-ringed protoplanetary disks. Astrophys. J. Lett. 869, L46 (2018).
Johansen, A. et al. Rapid planetesimal formation in turbulent circumstellar disks. Nature 448, 1022–1025 (2007).
Müller, J., Savvidou, S. & Bitsch, B. The water-ice line as a birthplace of planets: implications of a species-dependent dust fragmentation threshold. Astron. Astrophys. 650, A185 (2021).
Charnoz, S., Avice, G., Hyodo, R., Pignatale, F. C. & Chaussidon, M. Forming pressure traps at the snow line to isolate isotopic reservoirs in the absence of a planet. Astron. Astrophys. 652, A35 (2021).
Gundlach, B. & Blum, J. The stickiness of micrometer-sized water-ice particles. Astrophys. J. 798, 34 (2015).
Desch, S. J. & Turner, N. J. High-temperature ionization in protoplanetary disks. Astrophys. J. 811, 156 (2015).
Flock, M., Fromang, S., Turner, N. J. & Benisty, M. 3D radiation nonideal magnetohydrodynamical simulations of the inner rim in protoplanetary disks. Astrophys. J. 835, 230 (2017).
Qi, C. et al. Imaging of the CO snow line in a solar nebula analog. Science 341, 630–632 (2013).
van ’t Hoff, M. L. R., Walsh, C., Kama, M., Facchini, S. & van Dishoeck, E. F. Robustness of N2H+ as tracer of the CO snowline. Astron. Astrophys. 599, A101 (2017).
Pinilla, P. et al. Trapping dust particles in the outer regions of protoplanetary disks. Astron. Astrophys. 538, A114 (2012).
Dittrich, K., Klahr, H. & Johansen, A. Gravoturbulent planetesimal formation: the positive effect of long-lived zonal flows. Astrophys. J. 763, 117 (2013).
Izidoro, A., Bitsch, B. & Dasgupta, R. The effect of a strong pressure bump in the Sun’s natal disk: terrestrial planet formation via planetesimal accretion rather than pebble accretion. Astrophys. J. 915, 62 (2021).
Dra̧żkowska, J. & Alibert, Y. Planetesimal formation starts at the snow line. Astron. Astrophys. 608, A92 (2017).
Simon, J. B., Armitage, P. J., Li, R. & Youdin, A. N. The mass and size distribution of planetesimals formed by the streaming instability. I. The role of self-gravity. Astrophys. J. 822, 55 (2016).
Chambers, J. A semi-analytic model for oligarchic growth. Icarus 180, 496–513 (2006).
Tanaka, H., Takeuchi, T. & Ward, W. R. Three-dimensional interaction between a planet and an isothermal gaseous disk. I. Corotation and Lindblad torques and planet migration. Astrophys. J. 565, 1257–1274 (2002).
Lambrechts, M. et al. Formation of planetary systems by pebble accretion and migration. How the radial pebble flux determines a terrestrial-planet or super-Earth growth mode. Astron. Astrophys. 627, A83 (2019).
Hansen, B. M. S. Formation of the terrestrial planets from a narrow annulus. Astrophys. J. 703, 1131–1140 (2009).
Izidoro, A., Haghighipour, N., Winter, O. ~C. & Tsuchida, M. Terrestrial planet formation in a protoplanetary disk with a local mass depletion: a successful scenario for the formation of Mars. Astrophys. J. 782, 31 (2014).
Izidoro, A., Raymond, S. N., Morbidelli, A. & Winter, O. C. Terrestrial planet formation constrained by Mars and the structure of the asteroid belt. Mon. Not. R. Astron. Soc. 453, 3619–3634 (2015).
Levison, H. F., Kretke, K. A. & Duncan, M. J. Growing the gas-giant planets by the gradual accumulation of pebbles. Nature 524, 322–324 (2015).
Raymond, S. N. & Izidoro, A. The empty primordial asteroid belt. Sci. Adv. 3, e1701138 (2017).
Morbidelli, A. et al. Fossilized condensation lines in the Solar System protoplanetary disk. Icarus 267, 368–376 (2016).
Warren, P. H. Stable-isotopic anomalies and the accretionary assemblage of the Earth and Mars: a subordinate role for carbonaceous chondrites. Earth Planet. Sci. Lett. 311, 93–100 (2011).
Dauphas, N. et al. Calcium-48 isotopic anomalies in bulk chondrites and achondrites: evidence for a uniform isotopic reservoir in the inner protoplanetary disk. Earth Planet. Sci. Lett. 407, 96–108 (2014).
Wittmann, A. et al. Petrography and composition of Martian regolith breccia meteorite Northwest Africa 7475. Meteorit. Planet. Sci. 50, 326–352 (2015).
Lodders, K. An oxygen isotope mixing model for the accretion and composition of rocky planets. Space Sci. Rev. 92, 341–354 (2000).
Dauphas, N. The isotopic nature of the Earth’s accreting material through time. Nature 541, 521–524 (2017).
Brasser, R., Mojzsis, S. J., Matsumura, S. & Ida, S. The cool and distant formation of Mars. Earth Planet. Sci. Lett. 468, 85–93 (2017).
Bottke, W. F., Nesvorný, D., Grimm, R. E., Morbidelli, A. & O’Brien, D. P. Iron meteorites as remnants of planetesimals formed in the terrestrial planet region. Nature 439, 821–824 (2006).
Chambers, J. E. Making more terrestrial planets. Icarus 152, 205–224 (2001).
Kokubo, E. & Ida, S. Formation of protoplanet systems and diversity of planetary systems. Astrophys. J. 581, 666–680 (2002).
Bus, S. J. & Binzel, R. P. Phase II of the Small Main-Belt Asteroid Spectroscopic Survey. A feature-based taxonomy. Icarus 158, 146–177 (2002).
Urey, H. C. The cosmic abundances of potassium, uranium, and thorium and the heat balances of the Earth, the Moon, and Mars. Proc. Natl Acad. Sci. USA 41, 127–144 (1955).
Vernazza, P., Zanda, B., Nakamura, T., Scott, E. R. D. & Russell, S. In The Formation and Evolution of Ordinary Chondrite Parent Bodies (eds Bottke, W. F., DeMeo, F. E. & Michel, P.) 617–634 (University of Arizona Press, 2015).
Weiss, B. P. & Elkins-Tanton, L. T. Differentiated planetesimals and the parent bodies of chondrites. Annu. Rev. Earth Planet. Sci. 41, 529–560 (2013).
Neumann, W., Kruijer, T. S., Breuer, D. & Kleine, T. Multistage core formation in planetesimals revealed by numerical modeling and Hf–W chronometry of iron meteorites. J. Geophys. Res. Planets 123, 421–444 (2018).
Sanders, I. S. & Scott, E. R. D. The origin of chondrules and chondrites: debris from low-velocity impacts between molten planetesimals? Meteorit. Planet. Sci. 47, 2170–2192 (2012).
Moskovitz, N. & Gaidos, E. Differentiation of planetesimals and the thermal consequences of melt migration. Meteorit. Planet. Sci. 46, 903–918 (2011).
Asphaug, E., Jutzi, M. & Movshovitz, N. Chondrule formation during planetesimal accretion. Earth Planet. Sci. Lett. 308, 369–379 (2011).
Desch, S. J. & Connolly, J. H. C. A model of the thermal processing of particles in solar nebula shocks: application to the cooling rates of chondrules. Meteorit. Planet. Sci. 37, 183–207 (2002).
Yang, C. C., Johansen, A. & Carrera, D. Concentrating small particles in protoplanetary disks through the streaming instability. Astron. Astrophys. 606, A80 (2017).
Kunitomo, M., Guillot, T., Ida, S. & Takeuchi, T. Revisiting the pre-main-sequence evolution of stars. II. Consequences of planet formation on stellar surface composition. Astron. Astrophys. 618, A132 (2018).
Izidoro, A., Morbidelli, A., Raymond, S. N., Hersant, F. & Pierens, A. Accretion of Uranus and Neptune from inward-migrating planetary embryos blocked by Jupiter and Saturn. Astron. Astrophys. 582, A99 (2015).
Tsiganis, K., Gomes, R., Morbidelli, A. & Levison, H. F. Origin of the orbital architecture of the giant planets of the Solar System. Nature 435, 459–461 (2005).
Nesvorný, D. Dynamical evolution of the early Solar System. Annu. Rev. Astron. Astrophys. 56, 137–174 (2018).
Deienno, R., Morbidelli, A., Gomes, R. S. & Nesvorný, D. Constraining the giant planets’ initial configuration from their evolution: implications for the timing of the planetary instability. Astron. J. 153, 153 (2017).
Nesvorný, D. et al. OSSOS XX: the meaning of Kuiper Belt colors. Astron. J. 160, 46 (2020).
Gladman, B., Marsden, B. G. & Vanlaerhoven, C. In The Solar System Beyond Neptune (eds Barucci, M. A., Boehnhardt, H., Cruikshank, D. P. & Morbidelli, A.) 43–57 (University of Arizona Press, 2008).
Fressin, F. et al. The false positive rate of Kepler and the occurrence of planets. Astrophys. J. 766, 81 (2013).
Mayor, M. et al. The HARPS search for southern extra-solar planets XXXIV. Occurrence, mass distribution and orbital properties of super-Earths and Neptune-mass planets. Preprint at https://arxiv.org/abs/1109.2497 (2011).
Izidoro, A. et al. Formation of planetary systems by pebble accretion and migration. Hot super-Earth systems from breaking compact resonant chains. Astron. Astrophys. 650, A152 (2021).
Morbidelli, A. Planet formation by pebble accretion in ringed disks. Astron. Astrophys. 638, A1 (2020).
Lambrechts, M., Johansen, A. & Morbidelli, A. Separating gas-giant and ice-giant planets by halting pebble accretion. Astron. Astrophys. 572, A35 (2014).
Raymond, S. N., Izidoro, A. & Morbidelli, A. In Solar System Formation in the Context of Extra-Solar Planets inPlanetary Astrobiology (eds Meadows, V. S., Arney, G. N., Schmidt, B. E. & Des Marais, D. J.) (University of Arizona Press, 2020).
Pinilla, P., Pohl, A., Stammler, S. M. & Birnstiel, T. Dust density distribution and imaging analysis of different ice lines in protoplanetary disks. Astrophys. J. 845, 68 (2017).
Dra̧żkowska, J. & Dullemond, C. P. Planetesimal formation during protoplanetary disk buildup. Astron. Astrophys. 614, A62 (2018).
Ueda, T., Flock, M. & Okuzumi, S. Dust pileup at the dead-zone inner edge and implications for the disk shadow. Astrophys. J. 871, 10 (2019).
Ida, S., Guillot, T. & Morbidelli, A. The radial dependence of pebble accretion rates: a source of diversity in planetary systems. I. Analytical formulation. Astron. Astrophys. 591, A72 (2016).
Zhang, Y. & Jin, L. The evolution of the snow line in a protoplanetary disk. Astrophys. J. 802, 58 (2015).
Zhang, K., Blake, G. A. & Bergin, E. A. Evidence of fast pebble growth near condensation fronts in the HL Tau protoplanetary disk. Astrophys. J. Lett. 806, L7 (2015).
Baillié, K., Marques, J. & Piau, L. Building protoplanetary disks from the molecular cloud: redefining the disk timeline. Astron. Astrophys. 624, A93 (2019).
Bitsch, B., Morbidelli, A., Lega, E. & Crida, A. Stellar irradiated discs and implications on migration of embedded planets. II. Accreting-discs. Astron. Astrophys. 564, A135 (2014).
Ziampras, A., Ataiee, S., Kley, W., Dullemond, C. P. & Baruteau, C. The impact of planet wakes on the location and shape of the water ice line in a protoplanetary disk. Astron. Astrophys. 633, A29 (2020).
Birnstiel, T., Andrews, S. M., Pinilla, P. & Kama, M. Dust evolution can produce scattered light gaps in protoplanetary disks. Astrophys. J. Lett. 813, L14 (2015).
Drążkowska, J., Alibert, Y. & Moore, B. Close-in planetesimal formation by pile-up of drifting pebbles. Astron. Astrophys. 594, A105 (2016).
Asplund, M., Grevesse, N., Sauval, A. J. & Scott, P. The chemical composition of the Sun. Annu. Rev. Astron. Astrophys. 47, 481–522 (2009).
Desch, S. J., Kalyaan, A. & O’D. Alexander, C. M. The effect of Jupiter’s formation on the distribution of refractory elements and inclusions in meteorites. Astrophys. J. Suppl. Ser. 238, 11 (2018).
Pinilla, P., Lenz, C. T. & Stammler, S. M. Growing and trapping pebbles with fragile collisions of particles in protoplanetary disks. Astron. Astrophys. 645, A70 (2021).
Schneider, A. D. & Bitsch, B. How drifting and evaporating pebbles shape giant planets. I. Heavy element content and atmospheric C/O. Astron. Astrophys. 654, A71 (2021).
Lenz, C. T., Klahr, H., Birnstiel, T., Kretke, K. & Stammler, S. Constraining the parameter space for the solar nebula. The effect of disk properties on planetesimal formation. Astron. Astrophys. 640, A61 (2020).
Lenz, C. T., Klahr, H. & Birnstiel, T. Planetesimal population synthesis: pebble flux-regulated planetesimal formation. Astrophys. J. 874, 36 (2019).
Okuzumi, S. & Hirose, S. Planetesimal formation in magnetorotationally dead zones: critical dependence on the net vertical magnetic flux. Astrophys. J. Lett. 753, L8 (2012).
Lynden-Bell, D. & Pringle, J. E. The evolution of viscous discs and the origin of the nebular variables. Mon. Not. R. Astron. Soc. 168, 603–637 (1974).
Shakura, N. I. & Sunyaev, R. A. Black holes in binary systems. Observational appearance. Astron. Astrophys. 24, 337–355 (1973).
Bai, X.-N. & Stone, J. M. Magnetic flux concentration and zonal flows in magnetorotational instability turbulence. Astrophys. J. 796, 31 (2014).
Gerbig, K., Lenz, C. T. & Klahr, H. Linking planetesimal and dust content in protoplanetary disks via a local toy model. Astron. Astrophys. 629, A116 (2019).
Ormel, C. W. & Klahr, H. H. The effect of gas drag on the growth of protoplanets. Analytical expressions for the accretion of small bodies in laminar disks. Astron. Astrophys. 520, A43 (2010).
Johansen, A. & Lambrechts, M. Forming planets via pebble accretion. Annu. Rev. Earth Planet. Sci. 45, 359–387 (2017).
Walsh, K. J. & Levison, H. F. Planetesimals to terrestrial planets: collisional evolution amidst a dissipating gas disk. Icarus 329, 88–100 (2019).
Deienno, R., Walsh, K. J., Kretke, K. A. & Levison, H. F. Energy dissipation in large collisions—no change in planet formation outcomes. Astrophys. J. 876, 103 (2019).
Morbidelli, A. & Crida, A. The dynamics of Jupiter and Saturn in the gaseous protoplanetary disk. Icarus 191, 158–171 (2007).
Raymond, S. N., Quinn, T. & Lunine, J. I. Making other earths: dynamical simulations of terrestrial planet formation and water delivery. Icarus 168, 1–17 (2004).
O’Brien, D. P., Morbidelli, A. & Levison, H. F. Terrestrial planet formation with strong dynamical friction. Icarus 184, 39–58 (2006).
Levison, H. F., Duncan, M. J. & Thommes, E. A Lagrangian Integrator for Planetary Accretion and Dynamics (LIPAD). Astron. J. 144, 119 (2012).
Chambers, J. E. A hybrid symplectic integrator that permits close encounters between massive bodies. Mon. Not. R. Astron. Soc. 304, 793–799 (1999).
Try Adsterra Earnings, it’s 100% Authentic to make money more and more.
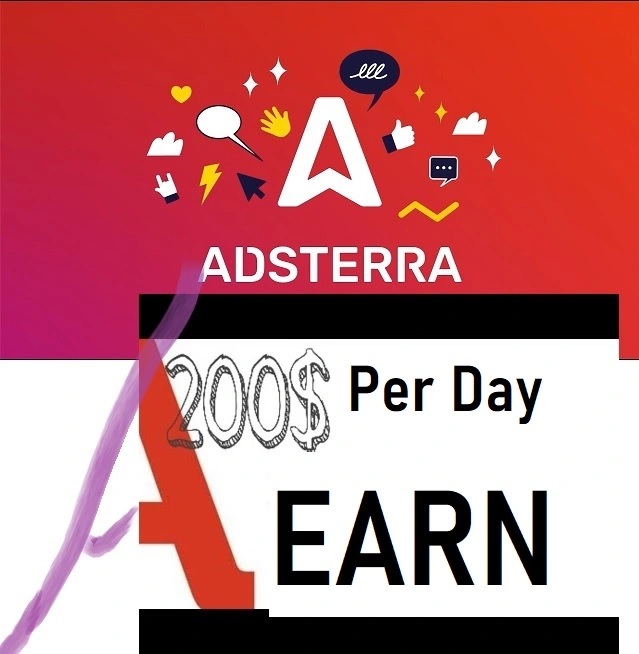
More Story on Source:
*here*
Planetesimal rings as the cause of the Solar System’s planetary architecture
Published By
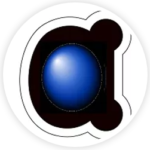
Latest entries
allPost2024.11.2310 Giros Dado sem Entreposto nos Melhores Cassinos Online Novembro 2024
allPost2024.11.23Giros Dado sobre Cassinos 2024 Top Ofertas uma vez que Free Spins
allPost2024.11.23Bónus BDM Bet Casino 450¤, 250 Rodadas Dado & Cashback
allPost2024.11.23Erlebe den Nervenkitzel von Casinia Casino – Jetzt anmelden und Bonus sichern!