Analysis of the Breakthrough Listen signal of interest blc1 with a technosignature verification framework
In parallel with the drift rate analysis, we performed a search for reappearances of the signal of interest on other days and at other frequencies.
Try Adsterra Earnings, it’s 100% Authentic to make money more and more.
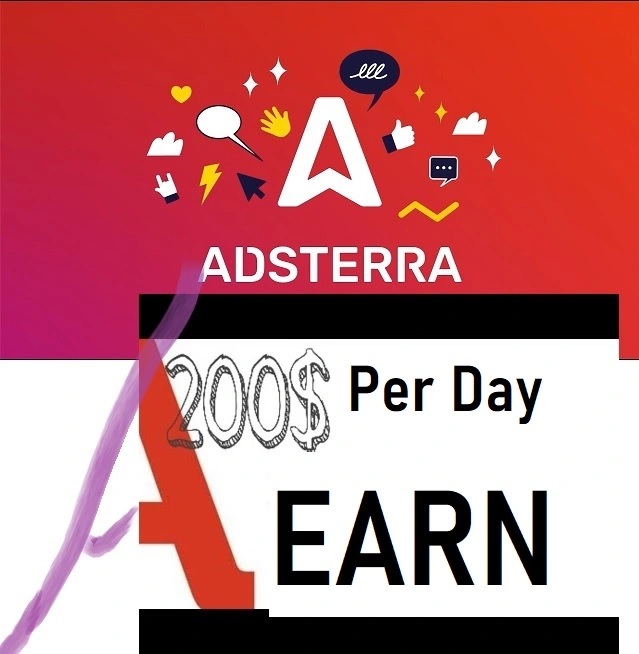
Other Murriyang signals near 982 MHz
We searched for signals with the same frequency and same drift as blc1 from both the week-long ProxCen campaign and every archival observation from standard BL Murriyang UWL observing of other stellar targets.
To find all appearances of blc1, even those that were too faint or too masked by RFI to be flagged by turboSETI, we produced output plots for visual inspection from every ProxCen on-source and off-source observation from April 29 to May 4. We restricted the plots to 982.002–982.004 MHz to begin with; a few plots were extended up or down by 1 kHz if there appeared to be interesting behaviour near the upper or lower bounds.
We created and searched two kinds of output plots, namely dynamic time–frequency spectra ‘waterfall’ plots (Fig. 1) and ‘butterfly’ plots, which display the power at each drift-rate–frequency pair after use of a de-drifting algorithm (Supplementary Methods 2.1). Examples of these output plots are shown in Supplementary Fig. 17.
Through analysis of these diagnostic plots, we identified four occurrences of blc1-like signals during the ProxCen observations, which, through their low S/Ns, had failed to reach turboSETI’s detection threshold. An example of one of these similar signals is shown in Fig. 3; the rest are displayed in Supplementary Figs. 3–5.
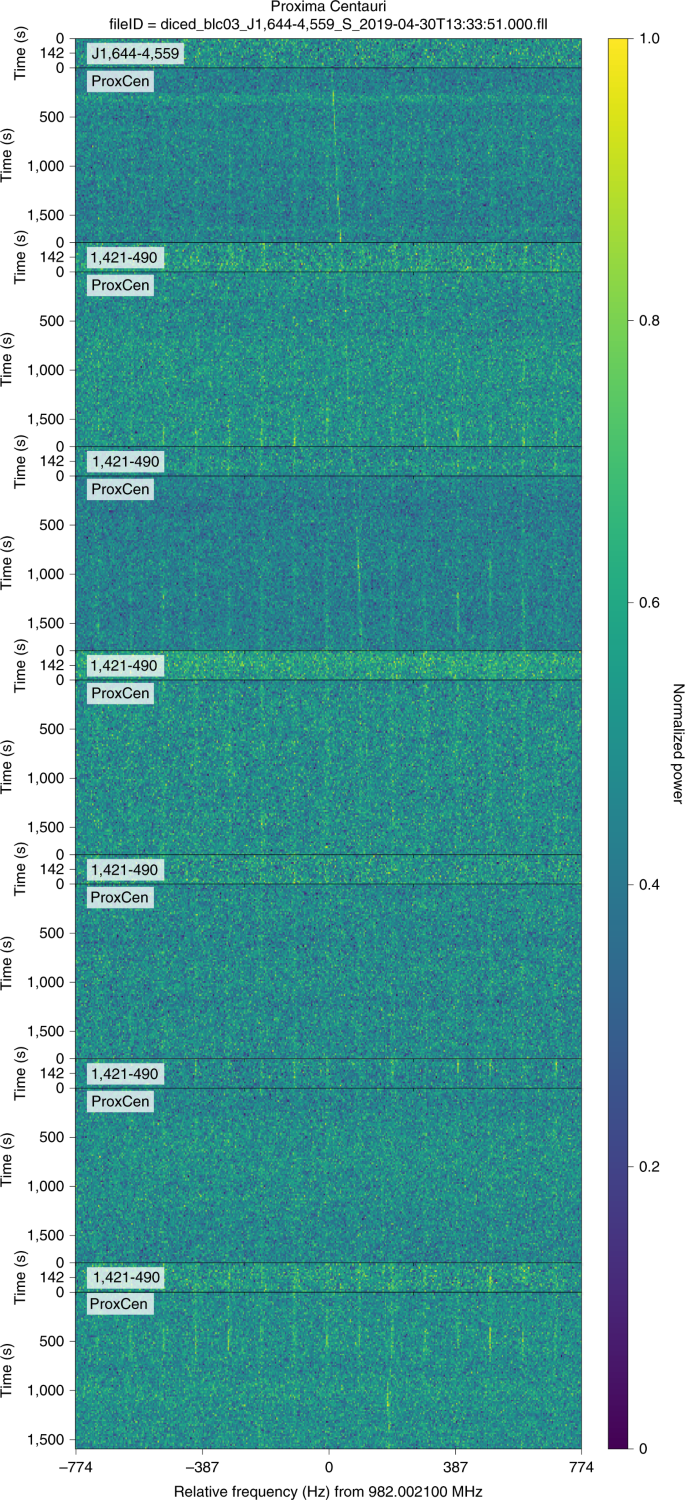
The signal is the bright sloping feature, but is clearly overlaid on a non-drifting, comb-like signal (see ‘Frequency comb’). Both the signal and the comb underneath seem to be RFI as they appear in the off-source observations. In Supplementary Table 1, we derived the S/N, drift rate and start frequency of each panel of the blc1 observation; we derived the properties of this signal in the same manner (Supplementary Methods 2.1). The drift rate (median 0.021 Hz s−1), S/N (median 6.9) and frequency range (982.0021–982.0023 MHz) are consistent with those of blc1, and this signal is also unresolved.
We performed the same analysis with every archival observation that BL had taken with the Murriyang UWL receiver. In total, this consisted of about 7,000 observations from 2019 to 2020, primarily nearby stars in the Hipparcos catalogue17,18 but also pulsars and quasars used for calibration and, of course, ProxCen. Most of these files had a standard duration of 5 min, as opposed to the 30 min observations from the ProxCen campaign. In many of these observations, we observed the same zero-drift frequency comb as detected in the original blc1 observation (see ‘Initial investigation and parametrization of blc1’), giving more evidence that this comb is unrelated RFI.
We found 15 similar-looking features in these non-ProxCen UWL observations. Visual inspection of the full cadences surrounding these features shows that 14 of them are different from the blc1-like signals in morphology, length, drift behaviour and/or signal strength over time. However, one of the 15 features is clearly RFI due to its persistence across both on-source and off-source observations, appeared four days prior to blc1 and looks to be potentially a member of the set including blc1 and the similar signals from the ProxCen campaign; this signal is shown in Supplementary Fig. 6.
All five of these 982 MHz signals from different days are fainter than blc1; three of them conclusively appear in the off-sources, whereas two of them are inconclusive.
Different frequencies
Human-made communication technologies often use multiple simultaneous frequency channels to send information for improved redundancy and bit rate. It is possible that extraterrestrial intelligence would do the same; it is also possible that the appearance of a blc1 twin at another frequency, if clearly RFI, would allow us to determine that blc1 is also RFI.
To find signals similar to blc1 at different frequencies, we calculated the frequency-normalized drift rate (({dot{nu }}_{mathrm{normalized}}=frac{dot{nu }}{nu })) that would indicate a signal that was drifting proportionally to blc1 in the first observation. This proportional drift is expected for multi-frequency transmitters in the same accelerational environment, but also in multi-frequency transmitters that are drifting electronically.
We then searched the catalogue of narrowband hits created with turboSETI for signals that appeared at the same time as blc1 and were drifting proportionally in the first panel, plus or minus the drift rate error proxy as given in the first row of Supplementary Table 1. This search returned 112 hits: blc1 itself and 111 signals that turboSETI had identified as hits but then rejected as RFI at an early stage in the pipeline due to their appearance in off-source observations. These hits were plotted in the context of all of the subsequent panels of the ProxCen observation on 2019 April 29.
We visually inspected the 111 matches, looking for signals that had the same morphology as that of blc1 beyond the drift rate in the first panel. To identify a signal with the same morphology, we looked for monotonicity, a shallowing of the slope over time, a vertical length that spanned multiple panels and the absence of complex additional features (for example sinusoidal behaviour). We found that 36 of the 111 turboSETI matches (32%) were blc1 ‘lookalikes’, namely signals with morphology strikingly similar to that of blc1. A subset of these lookalikes is shown in Supplementary Fig. 7, and some examples of the ‘non-lookalikes’ are shown in Supplementary Fig. 8.
The lookalikes have a range of variabilities over time, which seem to indicate multiple transmitters producing unusually consistent drifts. We then performed the same search as before but with negative drift rates. We found 310 hits from RFI, of which 27, upon visual inspection, were found to be mirrored lookalikes, with exactly the same drift structure over time as blc1 but flipped in morphology across the frequency axis. A selection of these signals is shown in Supplementary Fig. 9.
We can conclusively state that all lookalike and mirrored lookalike signals are RFI due to their appearance in off-source observations.
Characterizing the blc1 lookalike population
The presence of this population of both positively and negatively sloped blc1 lookalikes preliminarily suggests that all lookalikes (including blc1) share a common origin. We can further assess this claim by examining the similarity in the parametric distributions of the lookalike signals and of blc1. We find that blc1 is consistent with the lookalike population in absolute drift rate, frequency and S/N (Fig. 4).
Fig. 4: A corner plot showing the population of lookalikes and mirrored lookalikes compared to blc1 in start frequency, normalized drift rate and signal-to-noise ratio.
For the mirrored lookalikes, which have negative normalized drift rates, we took the absolute value for comparison. The heights of the kernel density estimations are not to scale, as the population of blc1 points is so much smaller than the other two populations that it would otherwise not be visible on these axes. The blc1 signal (x symbols and grey shading) is consistent with the signal-to-noise and normalized drift rate distributions (blue dots and shading for lookalikes, and red dots and shading for mirrored lookalikes) and, although slightly higher in frequency than the peak of the kernel density estimations for the lookalike population, is still consistent with that distribution.
Determining the origin of the blc1 lookalike population
We can further strengthen the claim that the lookalike population shares a common origin if we can identify a frequency-shifting instrumental or electronic effect in the data. One potential source of that effect is instrumental harmonic distortion, which can produce replicas of an original frequency f signal at 2f, 3f and so on. Another potential source is intermodulation distortion, a superset to harmonic distortion, which can produce a near-arbitrarily complex sequence of replicas that are integer multiples of the sums and differences of two or more original signals.
Harmonic analysis
We investigated whether any of the positive-drift lookalikes could be linked via a harmonic sequence. We generated the first 20 harmonics of a range of fundamental frequencies starting outside the bandpass at 100 MHz and progressing to 1,000 MHz in 1 kHz intervals. We defined a potential ‘harmonic sequence’ within the data as a set of two or more lookalikes (blc1 included) associated by the same fundamental frequency, within 1 kHz of their theoretical values. The blc1 signal was not consistent with being in a harmonic sequence with any of the observed lookalikes. However, a harmonic sequence did interlink a set of other lookalikes (Supplementary Table 2). This harmonic sequence contained frequencies of the form n + 0.1m + 0.099 MHz, where m and n are integers; because of the constant term, we refer to this set as ‘x.y99’.
Intermodulation analysis
Some lookalikes showed additional frequency structure that was not present in blc1. Two sets of lookalikes, which we will refer to as ‘triple feature’ (TF) and ‘single feature’ (SF), were distinguishable from their morphology alone. An example from the TF set is shown in Supplementary Fig. 10. TF and SF contained both positive lookalikes and mirrored lookalikes. TF had spacings that were integer multiples of 133.33 MHz. SF had a more complicated relationship of spacings, primarily integer multiples of 15 MHz but with an additional appearance of 128 MHz. In all cases within both sets, these frequencies are consistent with common clock oscillator frequencies used in digital electronics, with matches within 1–1,000 Hz of the expected value.
The three initially identified sets, namely TF, SF and the harmonic ‘x.y99’ set (see ‘Harmonic analysis’), each have a transition region in which the morphology of the signal flips, with positive lookalikes on one side and mirrored lookalikes on the other (Supplementary Fig. 11). If intermodulation effects were present, we predicted that we should detect a strong, zero-drift interferer at a frequency within the transition region, whose position is dictated by clock oscillator frequencies previously identified in the set.
For TF, we find a strong interferer at the predicted central frequency of 1,400 MHz, consistent to a single channel (±4 Hz). For SF, one central frequency consistent with the 30 MHz spacing is 1,200 MHz, where we also see a strong interferer consistent at the Hz level. For x.y99, we predict a central frequency of 1,332 MHz but see only an extremely faint interferer; we do see strong signals at 1,330.0000 MHz, 1,331.2000 MHz and 1,332.1805 MHz. This inconsistency could be caused by additional transposition (from an additional oscillator) present within the set, implying that one of the three listed frequencies is actually the responsible interferer. We conclude that these intentional-seeming separations seem likely to have been produced by the intermodulation of at least one clock oscillator with a strong interferer.
We found evidence for additional clock oscillator frequencies affecting the lookalike population, including the sets displayed in Fig. 5. As shown in Fig. 5a, we searched for patterns with integer kHz offsets (as in ‘Harmonic analysis’) and uncovered two individual sequences with spacings consistent with a 2.000004 MHz clock oscillator. Our data have a resolution of ~4 Hz, so we expect that the last digit has an error of ±4, which will propagate in the integer multiplication of spacings. In Fig. 5 we display the extra error at the Hz level to illustrate that these spacings are clearly the product of the same oscillator: not only are the general spacings consistent with powers of 2.000004 MHz, but the errors on those spacings are consistent with propagating errors on the order of ±4 Hz, for example, 16.000038 MHz. In Fig. 5b, we see these exact spacings relating blc1 (within propagated error, on the order of 100 Hz) with three lookalikes at ~712 MHz, ~856 MHz and ~1,062 MHz.
Fig. 5: A visualization of 16 signals from the lookalike set and blc1, divided into three sets.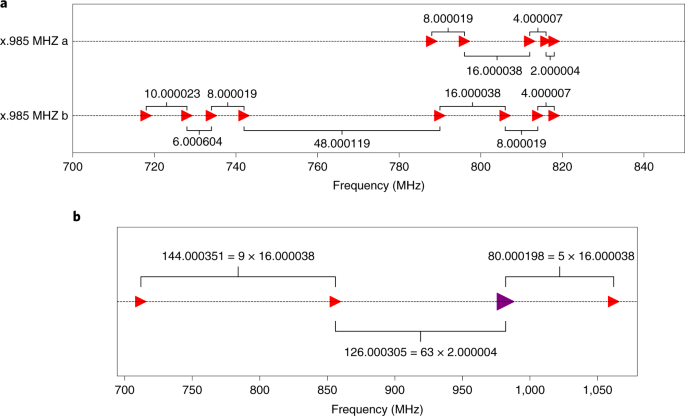
a, Two sets of positive lookalikes found within the data, originally identified by their position at 985 kHz above an integer MHz in each appearance. The full spacings, to Hz precision, are shown to demonstrate the set’s consistency with mixing from a 2.000004 MHz clock oscillator. Recall that the spacings have an inherent, multiplicative ±4 Hz error from the discrete frequency resolution. One spacing that we see in both sets, apparently resulting from this same oscillator, is 16.000038 MHz. The two sets are both affected by the same oscillator, but they are not consistent with each other to a multiple of 2.000004 MHz, illustrating another complexity within the data set. b, The blc1 signal (purple) shown in sequence with three additional lookalikes (red), which are consistent with integer multiples of 2.000004 MHz, including exactly 16.000038 MHz; this set of clock-oscillator-induced spacings is perfectly consistent with the spacings uncovered in the sets in a.
This numerical analysis indicates that blc1 is an intermodulation product produced by a ~2 MHz clock oscillator that is mixed with some other zero-drift RFI elsewhere in the band. We also find that power at the blc1-companion lookalike frequencies from Fig. 5 is detected when the four archival signals at 982.002 MHz are detected, just like blc1, and is not detected when the archival signals are not present (Supplementary Discussion 1.4).
This interpretation provides an explanation for the appearance of the signal in a part of the spectrum reserved for aviation and navigation: the source was not intending to transmit in that frequency region, and the signal was instead generated by the interaction of electronics within the transmitter, the receiver or both. In this case, the underlying signals that intermodulate to cause the lookalikes are likely from outside Murriyang’s receiver system. Signals are digitized using three analogue-to-digital cards inside the telescope focus cabin, with further processing done in radio-frequency-tight cabinets in the telescope tower6. As the lookalikes span across all three analogue-to-digital cards, they are unlikely to be spurious signals generated within the receiver’s digital systems.
The blc1 signal cannot be the original signal because it is two orders of magnitude weaker than the strongest signals in the set and is not seen in the off-panels, which is not replicated across the set. Evidently, blc1’s duty cycle or variability tracks the observing cadence on ProxCen, leading to the apparent localization on the sky. If blc1 were always ‘on’ at its brightest power, it would have been detected in all off-sources. Supplementary Figs. 7 and 9 reveal a range of inter-panel brightness behaviours for the lookalikes; some appeared in every panel, whereas some were as faint as blc1 and missing from all but the first panel. By the thresholding and on–off selection mechanisms, turboSETI selected the most interesting signal from a set of potentially hundreds of intermodulation products. These large numbers speak to the particular pathology of this case, as this behaviour had never been seen in over a year of UWL observations.
RFI environment analysis is unfortunately complex, and the RFI environment around most astronomical radio facilities is not well characterized at the frequency resolutions used for the SETI work. In the case of blc1, the situation is more complicated still, with mixing products that obscure the frequency and character of the original, individual interferers. It is possible that we could untangle the origins of this interference. However, as the goal of this study was to determine whether blc1 had an Earth-based or interstellar origin, we find that this is appropriate for future work.
More Story on Source:
*here*
Analysis of the Breakthrough Listen signal of interest blc1 with a technosignature verification framework
Published By
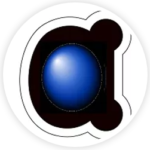
Latest entries
allPost2024.11.24243 Crystal Fruits Reversed Recensione Slot 2024 The National Music Conservatory
allPost2024.11.24Top Online Slots for Real Money in 2024: 10 Best Casino Sites
allPost2024.11.24Alistamento Infantilidade Pagamentos Como Principais Características Da Slot Machine Cash Pig
allPost2024.11.24Minutos Pagantes das Slots PG, Melhor Horário para Aprestar
50 replies on “Analysis of the Breakthrough Listen signal of interest blc1 with a technosignature verification framework”
Fantastic blog! Do you have any suggestions for aspiring writers? I’m planning to start my own site soon but I’m a little lost on everything. Would you propose starting with a free platform like WordPress or go for a paid option? There are so many choices out there that I’m totally confused .. Any suggestions? Kudos!
Thanks for sharing excellent informations. Your web site is very cool. I’m impressed by the details that you’ve on this site. It reveals how nicely you understand this subject. Bookmarked this web page, will come back for extra articles. You, my friend, ROCK! I found simply the information I already searched everywhere and just could not come across. What a great website.
Hello my friend! I wish to say that this post is amazing, nice written and come with almost all important infos. I’d like to peer more posts like this .
I feel that is among the such a lot vital information for me. And i’m satisfied reading your article. However wanna observation on some common issues, The website taste is great, the articles is actually excellent : D. Good activity, cheers
Aw, this was a really nice post. In concept I want to put in writing like this moreover – taking time and precise effort to make an excellent article… however what can I say… I procrastinate alot and under no circumstances seem to get something done.
Hi there, simply turned into alert to your blog via Google, and located that it’s truly informative. I’m gonna be careful for brussels. I’ll be grateful if you happen to continue this in future. Many folks will be benefited from your writing. Cheers!
I love your blog.. very nice colors & theme. Did you create this website yourself? Plz reply back as I’m looking to create my own blog and would like to know wheere u got this from. thanks
you are really a good webmaster. The site loading speed is amazing. It kind of feels that you are doing any unique trick. In addition, The contents are masterpiece. you have done a fantastic activity in this matter!
Renew is a nutritional supplement that activates your metabolism and promotes healthy sleep.
Today, I went to the beach front with my kids. I found a sea shell and gave it to my 4 year old daughter and said “You can hear the ocean if you put this to your ear.” She put the shell to her ear and screamed. There was a hermit crab inside and it pinched her ear. She never wants to go back! LoL I know this is completely off topic but I had to tell someone!
Hello my friend! I wish to say that this article is awesome, nice written and include approximately all significant infos. I¦d like to peer more posts like this .
Great – I should certainly pronounce, impressed with your web site. I had no trouble navigating through all tabs as well as related information ended up being truly easy to do to access. I recently found what I hoped for before you know it in the least. Reasonably unusual. Is likely to appreciate it for those who add forums or anything, website theme . a tones way for your client to communicate. Excellent task.
Way cool, some valid points! I appreciate you making this article available, the rest of the site is also high quality. Have a fun.
Hello. splendid job. I did not imagine this. This is a splendid story. Thanks!
You have observed very interesting points! ps nice site.
Hi my family member! I wish to say that this article is amazing, nice written and include almost all important infos. I would like to look more posts like this .
Very interesting info !Perfect just what I was looking for!
Hello, Neat post. There is an issue with your web site in web explorer, would test this… IE nonetheless is the marketplace chief and a huge part of other people will leave out your excellent writing due to this problem.
Good website! I really love how it is easy on my eyes and the data are well written. I am wondering how I could be notified when a new post has been made. I’ve subscribed to your RSS which must do the trick! Have a great day!
Hello I am so excited I found your website, I really found you by accident, while I was researching on Google for something else, Anyhow I am here now and would just like to say many thanks for a fantastic post and a all round entertaining blog (I also love the theme/design), I don’t have time to look over it all at the moment but I have saved it and also added your RSS feeds, so when I have time I will be back to read more, Please do keep up the great work.
Great ?V I should certainly pronounce, impressed with your website. I had no trouble navigating through all the tabs as well as related info ended up being truly easy to do to access. I recently found what I hoped for before you know it in the least. Quite unusual. Is likely to appreciate it for those who add forums or anything, site theme . a tones way for your client to communicate. Nice task..
This internet site is my breathing in, rattling superb design and perfect subject material.
You are a very bright individual!
Respect to website author, some good selective information.
Hello there, You have performed an incredible job. I will certainly digg it and personally suggest to my friends. I am sure they will be benefited from this web site.
Nice read, I just passed this onto a friend who was doing some research on that. And he actually bought me lunch because I found it for him smile So let me rephrase that: Thank you for lunch!
Does your website have a contact page? I’m having trouble locating it but, I’d like to shoot you an e-mail. I’ve got some suggestions for your blog you might be interested in hearing. Either way, great blog and I look forward to seeing it expand over time.
I was suggested this blog by my cousin. I am not sure whether this post is written by him as nobody else know such detailed about my trouble. You are wonderful! Thanks!
very good put up, i actually love this website, carry on it
Exactly what I was looking for, thanks for putting up.
What Is FitSpresso? The effective weight management formula FitSpresso is designed to inherently support weight loss. It is made using a synergistic blend of ingredients chosen especially for their metabolism-boosting and fat-burning features.
Today, while I was at work, my cousin stole my apple ipad and tested to see if it can survive a forty foot drop, just so she can be a youtube sensation. My iPad is now destroyed and she has 83 views. I know this is completely off topic but I had to share it with someone!
Magnificent site. Lots of useful information here. I am sending it to several friends ans also sharing in delicious. And obviously, thanks in your sweat!
Glad to be one of the visitors on this awing site : D.
In this awesome pattern of things you secure an A+ with regard to effort. Exactly where you actually confused us was on all the facts. You know, as the maxim goes, details make or break the argument.. And it could not be more true at this point. Having said that, let me say to you what did work. Your authoring is actually highly convincing which is most likely the reason why I am taking an effort in order to comment. I do not really make it a regular habit of doing that. Next, even though I can see a leaps in reason you come up with, I am definitely not confident of exactly how you seem to connect the details which in turn make the actual final result. For right now I shall subscribe to your point but wish in the foreseeable future you actually connect your facts much better.
I was recommended this website by my cousin. I’m not sure whether this post is written by him as nobody else know such detailed about my trouble. You are amazing! Thanks!
Enjoyed looking at this, very good stuff, regards. “A man may learn wisdom even from a foe.” by Aristophanes.
The next time I read a weblog, I hope that it doesnt disappoint me as much as this one. I imply, I do know it was my choice to read, however I actually thought youd have something attention-grabbing to say. All I hear is a bunch of whining about one thing that you could possibly fix when you werent too busy searching for attention.
I know this if off topic but I’m looking into starting my own blog and was curious what all is required to get setup? I’m assuming having a blog like yours would cost a pretty penny? I’m not very web smart so I’m not 100 certain. Any tips or advice would be greatly appreciated. Many thanks
As a Newbie, I am always searching online for articles that can aid me. Thank you
I view something genuinely interesting about your web blog so I bookmarked.
Lovely just what I was searching for.Thanks to the author for taking his time on this one.
You made some nice points there. I looked on the internet for the subject matter and found most people will agree with your blog.
I really enjoy looking at on this internet site, it has great blog posts. “Beware lest in your anxiety to avoid war you obtain a master.” by Demosthenes.
Rattling wonderful information can be found on website. “Many complain of their memory, few of their judgment.” by Benjamin Franklin.
But wanna comment that you have a very nice internet site, I like the design it actually stands out.
Great weblog here! Also your site quite a bit up fast! What web host are you using? Can I get your affiliate link on your host? I wish my site loaded up as quickly as yours lol
It?¦s actually a great and useful piece of info. I?¦m happy that you simply shared this useful info with us. Please stay us informed like this. Thank you for sharing.
Definitely believe that which you said. Your favorite justification seemed to be on the internet the simplest thing to be aware of. I say to you, I definitely get irked while people consider worries that they plainly do not know about. You managed to hit the nail upon the top as well as defined out the whole thing without having side effect , people can take a signal. Will probably be back to get more. Thanks
You should participate in a contest for the most effective blogs on the web. I’ll advocate this site!